Introduction
Over the past decade, archaeology and epigraphy have been reconsidering their modus operandi. Prompted and facilitated by technological advances, motivated by new research questions, and challenged by growing calls to engage with contemporary audiences, they have been experimenting with methodological approaches and interdisciplinary collaborations. Within this context, the Digital Epigraphy and Archaeology project (DEA) has been developing 3D digitization techniques that accommodate various types of artifacts, has been incorporating multidisciplinary approaches to achieve a more holistic stance towards the objects of study, and has focused on the reproducibility and accessibility of both its techniques and the 3D models.
The methods developed by the DEA project (www.digitalepigraphy.org) have been widely adopted and used so far in several projects, including the digitization of the collection of squeezes from the Monumentum Ancyranum, a collection of Abraham Lincoln’s Letters housed at the Library of Congress, collections of squeezes from Thasos housed at the University of Lyon 2, the Rosetta Stone at the British Museum, and several other projects that required different configuration of methods and accommodation of needs both in terms of the collections and the researchers.
This paper presents the DEA’s introspective and reembodied ways of preserving and studying the past by reconsidering historical artifacts and their digital re-materialization. The following sections discuss the project’s approach to copies and digital copies, 3D digitization and enhanced visualization processes, comprehensive cloud services, and 3D printing to present the DEA steps toward facilitating and advancing archaeology and epigraphy. Through such approaches that combine traditional rigor with technological novelty and affordances, the team’s vision is to popularize archaeology and epigraphy within and beyond academia and pinpoint the significance of the world’s heritage to the new generations of students and the public.
Authenticity and authenticity of experience
Appreciations of authenticity
and the art of copying
Ancient Greco-Roman inscriptions were engraved on stones, temple walls, funerary monuments, and objects of everyday use. They documented life and death as part of life, events, and historical milestones. Since then, they have been a living record. The first archaeologists, anxious to save the fleetingness of history, copied them on sketchbooks and made copies of them on paper and latex (known as squeezes). Later, photography facilitated their work and preserved a copy of those vanished(-ing) worlds. Still, the written word is only accessible to a few if it has not been destroyed altogether. So, giving access to this treasure trove of information will enable everyone to better understand the significance of archaeology and epigraphy for studying ancient societies.
Epigraphic squeezes, essentially being copies of the originals, may raise questions about their importance compared to the actual stone inscription. Still, their significance is undeniable, hence the need for their close study and preservation. Nonetheless, when we add to these considerations their 3D reproduction in the form of a digital squeeze, we need to address questions, such as: “Can the 3D model of the squeeze re-embody the real artifact, or is it a poor copy that cannot compare let alone rival the original?” And “What do we envision the impact of a 3D model to be when we move beyond its technological novelty?”
This section discusses authenticity as a concept and practicality in an attempt to create a framework for the appreciation of squeezes and digital squeezes. So, we begin with the fundamental question of how to determine authenticity. Below, we relay two diametrically opposing views on the subject—the debate resting on the monolithic and intransigent or the malleable and discursive.
“The authenticity of a thing is the essence of all that is transmissible from its beginning, ranging from its substantive duration to its testimony to the history which it has experienced. Since the historical testimony rests on the authenticity, the former, too, is jeopardized by reproduction when substantive duration ceases to matter. And what is really jeopardized when the historical testimony is affected is the authenticity of the object.” (Benjamin 2010)
“Beyond the cult of the valuable object… the predominant element in these museums, much more than the object in itself, is the discourse – the logical sequence, the syllogistic chain, the reasoning process which each individual display and the overall script of the exhibition as a whole seek to expound.” (Montaner 1990, 18-21)
The issue of copying is not new. Ancient Roman sculptors copied Greek originals. Are they any less valuable now? Do they have less to convey, or do they simply convey something else—different yet equally meaningful?1 The debate regarding originality and imitation is not new. Aristotle and Plato were the first to consider imitation and its value. Aristotle (Aristot., Poet., 1448b; Rhet. 1370a) thought it a worthy pursuit, an elevated action that validated the original and its creator. Plato (Plat., Rep., 602d-603e; 605a-c) famously disagreed and relegated imitation to the realm of poorness of thought.2 In literature, the period of the Second Sophistic was the time of reinventing the classical canon in an utterly innovative manner that encompassed imperial socio-cultural dynamics. Dionysius of Halicarnassus in On Imitation and Longinus in On the Sublime suggest sublimity through imitation and emulation. From the Roman perspective, Cicero suggests that Brutus imitates Cato but also improves on his work, changing the latter’s rougher words (horridiora verba, Brut. 68). And Quintilian considers imitation an intrinsic part of the learning process (Inst., 2.2.8; 2.4.12). Similarly, scholarship tended to judge the imitative spirit of the Second Sophistic harshly, and it was not until relatively recently that scholars were able to appreciate the uniqueness of imitation, both as a purveyor of the original and an original on its own accord.3
The artifact
When it comes to material culture, one can understand the seminal significance of the artifact, its value, aura, material, and constructional details that a copy cannot match.4 However, the situation is more complex when we consider the fragility of historical objects that limits their accessibility, their potential weathering or loss over time, or even the simple description of the field of archaeology as one of destruction—once the field is excavated, its originality is violated and ultimately destroyed.5
Beyond the perils of destruction, though, there are other parameters to consider. The goal of studying the past is to share this knowledge with younger generations and make it accessible to the global community. So, any effort to reproduce ancient art to make it approachable to students and the public is worth pursuing and retroactively again validates and reconfirms the importance of the field.
An example closer to our times and the discussion of artifacts and their accessibility is the Next Rembrandt Project (https://www.nextrembrandt.com/). A team of art scholars, museum experts, and computer scientists collaborated to train a computer in Rembrandt’s techniques and mannerisms. Their goal was to create paintings resembling the master’s handwork. The attempt has received mixed reviews, and one can understand both the excitement and the quibbles. Starting with the latter, reactions included apprehension at relegating the mastery of a genius to a soulless machine, endangering the creative process, and undermining the art world by insinuating copies that can very easily deceive.
“What a horrible, tasteless, insensitive and soulless travesty of all that is creative in human nature. What a vile product of our strange time when the best brains dedicate themselves to the stupidest ‘challenges’, when technology is used for things it should never be used for and everybody feels obliged to applaud the heartless results because so revere everything digital…”(Jones, The Guardian 2016)6
Conversely, individuals who are visually impaired and need to touch a piece of art to “see” it appreciated similar successful attempts to make the world’s treasures touchable and approachable to more.7 In such cases, the issue of accessibility takes a new meaning, as we are not debating the singularity of the artifact and the hubris of technological advances. Instead, we are faced with the reality that archaeology and epigraphy exclude a number of people whose only chance to “see” the artifacts is via their copies.8
“This blind man, an old friend of my wife’s, he was on his way to spend the night…Something about the church and the Middle Ages was on the TV…the TV showed this one cathedral…Then something occurred to me and I said: ‘Do you have any idea what a Cathedral is?’…‘Cathedrals’ the blind man said… ‘If you want the truth, bub, that’s about all I know…But maybe you could describe one to me?’” (Carver 1989, 209, 222-224)
Therefore, it is evident that reproducibility and its effect on the perception of authenticity are more complex than simply assigning polarizing black-and-white terms. We could argue that the value of any artifact and its historical significance only increases when it becomes a shared world heritage object valued by many. Working within this new mental and research framework, instead of scholars monopolizing the fields and subsequently struggling to explain their significance, archaeology and epigraphy can become a matter of public interest and concern.
Digital Epigraphy: a new version of epigraphy
as a newfound re-embodiment
Squeeze and digital squeeze:
an approved copy and its copy
Squeezes have always answered the call for preservation, dissemination, and tactility of inscriptions, as those involved found a way to re-embody the inscribed text and carry it with them. Also, as inscriptions undergo an expected level of wear and tear in addition to cases when dramatic changes in the geopolitical landscape jeopardize their existence, squeezes can be elevated into the only reliable and remaining descendants of the stone inscriptions. To that effect, Meskell very astutely observes:
“This trend of increasing heritage destruction uncomfortably suggests that there is something specific about the World Heritage stamp that has rendered sites valuable targets. Their imputed protected legal status under international treaties, the resources channeled to them, their visibility and symbolic value as the purported ‘heritage of humanity,’ and the outrage of the international community at their targeting have attracted new modalities of danger.” (Meskell 2018, 192)
With this in view, the DEA project focuses on the 3D digitization, preservation, accessibility, and dissemination of the squeeze in the form of a digital squeeze. The team has designed novel algorithms that can capture the 3D information contained on the surface of an epigraphic squeeze, using a minimal setup that utilizes accessible technologies, such as regular flatbed office scanners or digital cameras. Such a setup makes the digitization process affordable and practical for epigraphists and archaeologists to use and integrate within their existing workflow. Furthermore, the use of modern web technologies facilitates the storage and dissemination of the 3D digital squeezes on the cloud without additional software requirements or technical expertise from the end users who can easily access and seamlessly study the inscriptions from mainstream devices like computers, tablets, and smartphones.
The Digital Epigraphy and Archaeology Project
Specifically, the DEA project provides novel and technologically advanced scientific tools for the effective study and comparative analysis of inscriptions and other artifacts. It provides archaeologists and epigraphists with cost-effective and efficient methods for 3D digitization and options for enhanced visualizations and further automatic analyses.
The most popular and widely used DEA technology is the shape-from-shading (SFS) tool, which provides a framework to digitize squeezes with the use of an office scanner. The SFS tool runs as a web application focusing on digitizing, 3D visualization, data mining, and electronic dissemination of artifacts. The tridimensional digitization is achieved through the bidirectional scanning of the squeeze using a typical 2D office scanner. The scanned images are then processed by the SFS algorithm, which analyses the depicted shading in the images and captures in 3D the surface of the scanned squeeze. The advantages are numerous: 1) The process requires no expensive equipment. 2) The squeeze can be safely preserved in a digitized form, thus eliminating the possibility of deterioration of the squeezed paper. 3) The squeezes can also be distributed electronically, facilitating epigraphic studies. 4) Finally, the digital squeezes can be more effectively visualized compared to the 2D images, as they can be viewed from different angles and under different artificial lighting conditions.
The first version of the SFS tool was released to the public in 20129 with generous support from a start-up grant from the US National Endowment for the Humanities.10 The toolbox was implemented in Java as a software application that could be downloaded and run on any computer with Java Runtime Environment installed. The software could process images of scanned squeezes, reconstruct the 3D model of the squeeze, and instantly upload it to the digitalepigraphy.org server for web-based visualization and dissemination. Many research universities adopted the SFS tool to facilitate their squeeze digitization projects, such as Cornell University for the digitization of the squeezes from the Monumentum Ancyranum,11 the University of Lyon 2 for the digitization of the squeezes from the HiSoMA laboratory,12 and the digitization of the squeezes from the Ca’ Foscari University of Venice,13 among others. The total volume of tens of thousands of 3D digitized squeezes produced by these projects familiarized the epigraphic community with the newly formed concept of the digital squeeze and its advantages, as well as the best practices for digitizing and organizing them into databases.
The Digital Squeeze
To define the concept of the digital squeeze, we first need to identify the characteristics of the traditional epigraphic squeezes that need to be maintained in the digital form and then pinpoint which additional features can be added to enhance their use. An epigraphic squeeze can accurately document the details of a flat inscribed surface in the form of an embossed paper replica of the original surface. Creating a squeeze requires only paper material; therefore, it is cost-effective and easy to handle (acquire, prepare, process, carry, and store). However, despite the many advantages of the usability and ease of use of squeezes, there are also many limitations. Due to the flat nature of the paper, squeezes cannot easily capture non-flat objects, such as round surfaces, or multiple sides of a larger object at once. Furthermore, the size of the squeeze is limited by the size of the paper, which may be smaller than the size of the raw inscription, and in such cases, multiple squeezes are required to capture the entire surface. Finally, although squeezes can be easily stored and carried as rolls, the soft nature of the material makes them rather sensitive to pressure and other environmental conditions, such as humidity or dryness. As a result, the embossed details may be flattened over time, and the paper itself can deteriorate.
When comparing the paper squeeze with the digital and considering the latter’s possibilities, our team maintains that the digital equivalent of an epigraphic squeeze should be at least of the same quality or even superior, in terms of captured details, compared to the paper squeeze. Acquiring a digital squeeze must also be as easy and affordable as the traditional process. Also, the data need to be readily captured and processed within a few minutes and easily viewed, copied, and transferred. In terms of the current technology, this is translated to a sampling distance of at most 170 µm or spatial resolution of at least 150 DPI, which is equivalent to a squeeze of size 67.7 cm×50.8 cm (an area covered by 5.5 A4 pages) captured with a conventional 12 Megapixel digital camera sensor (4000×3000 pixels). The same camera can be used to digitize smaller inscriptions (33.8 cm×25.4 cm, an area slightly larger than an A4 page) at even higher resolutions (85 µm, 300 DPI). The camera resolution of modern smartphones exceeds the above specifications, making image-based techniques such as SFS accessible and cost-effective in terms of technological requirements. Obviously, the use of higher resolution camera sensors such as 48 Megapixels (iPhone 14 Pro) allows for even smaller sampling distance (85 µm instead of 170 µm) or larger digitized surfaces, which in this case is equivalent to a squeeze of size 135.4 cm×101.6 cm or an area covered by 22 A4 pages.
Furthermore, the ease of use and handling of a digital squeeze must be at least comparable to the convenience of a traditional paper squeeze, which in the digital case, is translated to small file sizes and convenient file formats. Based on the resolutions above, a photograph taken by a 12 MP camera can be stored in a file of size 3.6 MB using JPEG format at 100% quality compression, and in the case of a 48 MP camera, the size is 14.4 MB. The SFS algorithm requires 2 or 4 photos of the inscription or the paper squeeze taken from the same point of view under different lighting orientations.14 The result of the 3D digitization process is a depth map and an orientation map (also known as a normal map),15 which are images of the same size as the original photographs. These renderings capture the 3D information contained in the depicted artifact in a way similar to how a paper squeeze captures the details of a raw inscription. So, even if we account for the size of all input and output files together, the size of a 3D digital squeeze in terms of digital storage is equivalent to the size of 6 pictures at the most, which is about 20 MB, in the most typical scenario. Files of this size can be easily stored in low-cost digital storage or conveniently transferred over the internet. For example, a low-cost 128 GB USB thumb drive can store more than 6000 3D digital squeezes, and an internet connection with downloading speed of 300 Mbps can transfer a 3D squeeze at about half a second, which is fast enough for real-time browsing through an extensive collection of digital squeezes. The above numbers clearly demonstrate that, from many aspects, the ease of use of a digital squeeze is at least comparable to the convenience of a traditional paper squeeze.
However, a digital squeeze has many additional advantages that make it significantly superior to the paper one. First, the 3D details of a digital squeeze cannot deteriorate over time and can be easily stored in a minimal digital medium without requiring sizeable physical storage space. The digital squeeze can be viewed and studied conveniently, such as on a tablet computer, and interactively relit by reorienting the tablet with respect to a virtual light source.16 Furthermore, it can be visualized with different shading schemes to highlight various geometric features of the inscribed surface.17 The depth map and the edge map are two examples of such visualizations that can be used to better present the inscribed content compared to a regular photograph of the original object. Therefore, a digital squeeze can also be used to produce better illustrations for scholarly publications in the fields of epigraphy and archaeology. Finally, one crucial characteristic of the digital squeezes is that they can capture both raw inscriptions and paper squeezes because the method can be seamlessly applied to photographs of raw inscribed objects as well as paper squeezes. Therefore, digital squeezes can be used for the digital preservation of both types of artifacts in addition to many others, such as paper embossments, seals, casts of coins, and other flat inscribed or embossed surfaces.
The above discussion and observations have clearly defined the concept of the digital squeeze and formed our expectations about its affordances, which our team took under consideration when developing the most recent release of our digitization software.
Shape-from-shading software
as a publicly available cloud service
In 2022, 10 years after the release of the first version of the SFS toolbox and after accumulating a decade-long experience in 3D digitization of a large variety of artifacts of different sizes, materials, and conditions, we released a cloud-based version of the algorithm that significantly improves the digitization process, the dissemination of the digital squeezes, and the overall user experience (fig. 1). One of the key improvements is that the application is available across devices through a web browser and without the need to install additional software, a significant advantage over the previous version of the software. Furthermore, the users have access to cloud storage provided by the University of Florida,18 which is integrated with the SFS toolbox, to store the digital squeezes and organize them into collections. To access the cloud service, the users can log in with institutional or personal accounts through third-party authentication providers using state-of-the-art information technology protocols, such as the reliable JSON Web Token (JWT) exchange mechanism. Users from universities and research institutes that utilize such services can automatically login with their institutional email accounts through a single sign-on (SSO) protocol without the need to create separate accounts for this software. Once they log in, the users can organize their digitization projects in collections or batches of digital squeezes through cloud folders, similarly to how paper squeezes are cataloged and organized in physical storage. The software guides the users through the process of creating a digital squeeze, which involves three steps: 1) uploading the images of the artifact, 2) aligning the images, and 3) running the SFS algorithm.
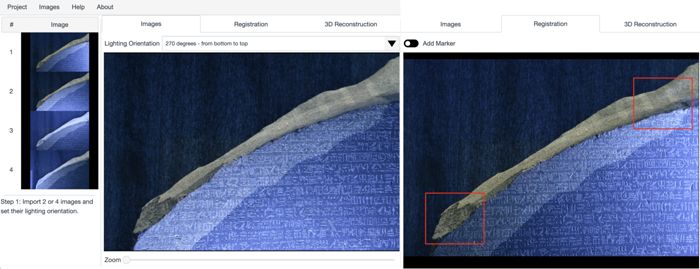
using custom markers shown here as red squares.
The input images can be either photographs taken from a stationary camera or images acquired from a flatbed scanner, depending on the type and size of the artifact. For example, smaller objects, such as paper squeezes, and casts of coins can be easily placed on a typical office scanner and scanned 2 or 4 times by rotating them 90 degrees between the scans. This process produces images of the same artifact under different lighting directions. The shading differences between these images capture the fine tridimensional details analyzed by the SFS algorithm to produce the 3D digital squeeze. A similar process can be followed for larger objects, such as raw inscriptions, using a fixed camera and a portable light source to illuminate the object from the top, bottom, left, and right to take the respective 4 pictures. After uploading the images and identifying the corresponding lighting directions, the user can align them with a manual registration process and finally perform the 3D reconstruction by running the SFS algorithm.
The produced digital squeeze is saved on the cloud and linked to its input images, the reconstructed depth map, the normal map, and their associated metadata (fig. 2). This comprehensive record of the digital squeeze allows reproducibility, an essential component of the modern research process that enables other scholars to repeat or even improve the 3D reconstruction results in the future. Another benefit of this integrated cloud record is that it can be viewed with different visualizations that combine the original image data and the 3D reconstructed surface. Our software provides a web-based 3D viewer that contains several options for manipulating and visualizing the digital squeeze, such as the depth map, a grey-scale visualization that highlights the deeper inscribed areas of the surface, and the edge map that highlights the curvature of the surface (fig. 3). The contrast between the brighter and darker areas in these maps gives better readings of the inscribed surface by intensifying the shape of the inscribed letters.
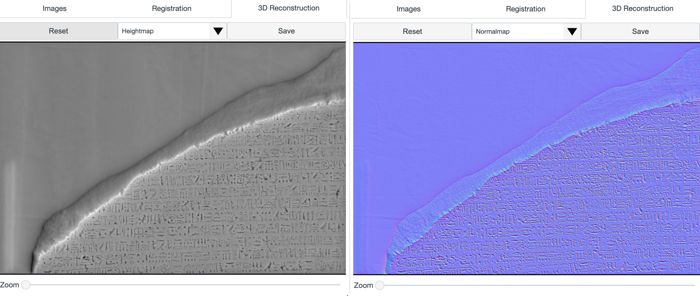
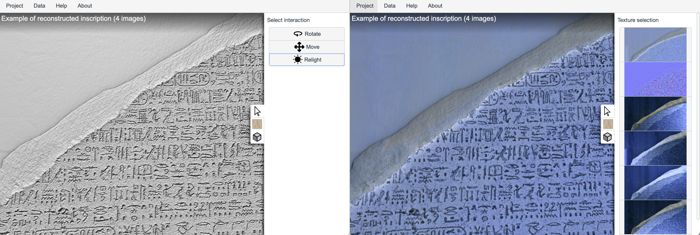
the digital squeeze better.
Finally, given the multitude of electronic devices available and the need to accommodate different audiences and users with varying needs, use-case scenarios, skillsets, and financial means, our team has devoted significant efforts so that the SFS toolbox and the 3D viewer can run on any mainstream web browser and a plethora of devices, including desktop computers, tablets, and smartphones. These devices can easily access a digital squeeze or collection of squeezes through a unique URL generated for each squeeze, which can be shared with others, can be embedded in online databases and curatorial software, or even disseminated through social platforms and learning management systems.
Copy vs. Original:
how a copy verifies the original
This section presents two case studies that demonstrate the process of making digital squeezes from a raw inscription and a paper squeeze.
An interesting case study is that of the Rosetta Stone housed at the British Museum. Undeniably one of the most famous artifacts, yet understandably so untouchable and over the past several decades unreadable in many parts. Our team successfully produced a high-resolution 3D digital squeeze based on photographs we took using 4 different lighting directions. We then processed it with our SFS algorithm, increasing its legibility and advancing the dissemination of its contents.19
The Digital Rosetta Stone project is a collaboration between the University of Leipzig, the Saxon Academy of Sciences and Humanities, the British Museum, and the University of Florida. For the 3D digitization, we extended the DEA’s previous methods to accommodate an artifact of this material, size, and placement by taking a series of photographs under controlled lighting and processing them to produce the first 3D digital squeeze in such high resolution and publish it as an open access resource.20 In our setup, we fixed a DSLR camera (Nikon D3400) on a tripod in front of the inscription. We used a hand-held lightsaber-type light source to illuminate the inscription from the top, bottom, left, and right to take photographs under 4 different lighting directions. By extending our algorithm to handle 4 lighting directions instead of 2, which was the requirement in the previous version, it can better separate the chromatic information from the structural information and handle non-uniform lighting distribution caused by the manual handling of the light source.
To assess the quality of the produced 3D digital squeeze, we identified several publicly available photographs and 3D models of the Rosetta Stone, which we use here as a benchmark. Fig. 4 shows a visual comparison of our digital squeeze with two 3D models of the Rosetta Stone by Jon Beck21 and the British Museum.22 To the best of our knowledge, these were the only publicly available 3D models of the Rosetta Stone at the time of publication. The 3D digital squeeze produced from our SFS tool demonstrates sharper details that significantly improve the legibility of the inscription compared to the other 3D models. Furthermore, we used as additional benchmarks two readable, high-contrast photographs of the inscription by the Wikipedia article on the Rosetta Stone23 and the British Museum online collection,24 both commonly referenced online resources on the artifact. Fig. 5 compares the depth map of our digital squeeze with these benchmarks. Based on this comparison, it is evident that the clarity and detail of the depth map25 are superior to the other images, and it is further demonstrated in fig. 6 by a side-by-side comparison of symbol 39, in line 11 of plate 6 (Sharpe 1871). The comparison also includes the hand-drawn illustration by Samuel Sharpe,26 which we consider here as the expert’s benchmark. From a simple observation, it is evident that the depth map of our digital squeeze closely resembles the original illustration, and the amount of detail captured in our digital squeeze exceeds the quality of the other images.
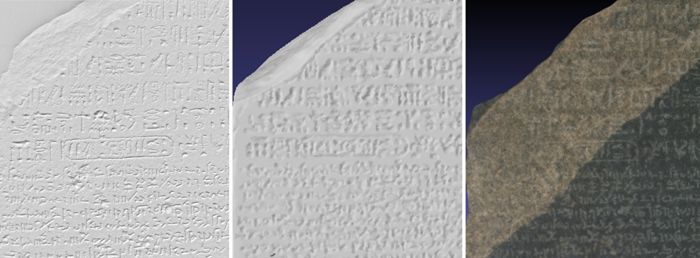
Right: The 3D model published by the British Museum in 2016.
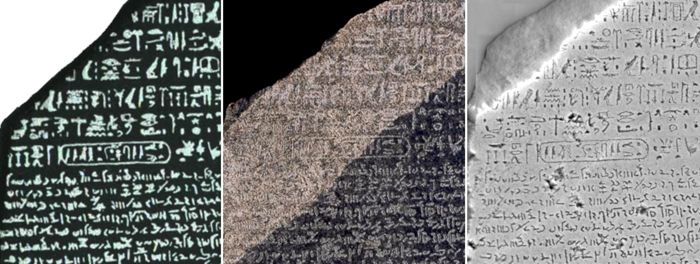

In sum, our goal was to create a visually enhanced 3D model of an artifact that is challenging in its physical positionality and readability and offer the scholars and the public enhanced engagement opportunities. Furthermore, such an attempt showcases the (re)constructive power of technological advances and the educational and research potential that such an integrated project can yield.
Finally, another case study showcasing our SFS method’s use is the 3D digitization of the squeezes from the Monumentum Ancyranum in collaboration with Cornell University. This collection contains squeezes from the expedition that the university had launched to Asia Minor and the Assyro-Babylonian Orient in 1907-1908. At the beginning of the expedition, team members spent two weeks creating squeezes of the Res Gestae of the emperor Augustus inscribed on the walls of the Monumentum Ancyranum. The squeezes traveled back to Cornell and have been housed there ever since, weathered by environmental conditions, humidity, dryness, and tear. The squeezes are large (greater than 1 meter in length) and, therefore, cannot be easily scanned on a typical flatbed office scanner. For this project, we used the scanner Super6k manufactured by Better Light, which is ideal for larger scanning jobs like this. The scanning process was repeated 4 times using an external light source on the top, bottom, left, and right side of the squeeze, respectively.
One of the scanned photographs from the collection of the digitized squeezes is shown in fig. 7. The squeeze is from the Greek Column 18, lines 17-24 of the Res Gestae Divi Augusti inscription. The size of the squeeze is 111.13 cm×53.18 cm and was scanned at 162 DPI, producing images of size 7088 px×3392 px. The 3D digital squeeze produced by our SFS tool is shown in fig. 8. Fig. 9 shows the edge map visualization of the digital squeeze, highlighting the inscribed edges.

Greek Column 18; Lines 17-24 of the Res Gestae Divi Augusti inscription from Monumentum Ancyranum.
Making the tangible intangible and vice versa:
3D printing and augmented reality
Thus far, the discussion has focused on the need to advance the opportunities for enhanced interaction with squeezes and the affordances of digital copies. However, another equally important aspect of tangibility is the physicality of the artifact, or its copy, for that matter. So, how does a digital initiative that advocates for more accessibility can contribute to that?
Our project works with 3D printing, essentially rematerializing the digital file into a tangible copy of the inscription in an attempt to physically re-embody the inscriptions and provide students and researchers with more meaningful experiences that materialize tactility. Additionally, we employ augmented reality devices that can virtually bring the artifacts into one’s workspace. Such an opportunity enhances the research and pedagogical experiences, as the user can “see” and interact with the artifacts in their own “physical” space.27
Experiential learning and its contribution to pedagogy is a well-studied research area (Alibali & Nathan 2009; Eisenberg & Pares 2014, 344-8; Goldin-Meadow 1999). Also, several studies have explored the significance of embodiment to learning (Abrahamson & Lindgren 2014; Eisenberg & Pares 2014, 347-8; Goldin-Meadow 2009). Additionally, mixed-reality environments have been shown to enrich educational experiences, leading to better learning outcomes (Lindgren & Johnson-Glenberg 2013).
Within this framework of a reembodied pedagogy, the DEA project works toward the re-physicalization of the digital copy through 3D printing. In this case, utilizing the advantages of the digital squeeze and the unique way it preserves the original stone inscription, we are bringing it back to human hands as an object that can now be touched and studied safely and anew. These new 3D-printed models are new objects that can assist more people in realizing the authenticity of the originals, as they afford accessibility that may lead to closer study and scrutiny of details, physical contact that is otherwise prohibitive, and the ability to provide new experiences to people. Our hope is that by re-incorporating inscriptions in a tangible form into our research, teaching, and everyday life, we will engage new audiences and animate new authentic user experiences.
To this end, our team works with the Marston Science Libraries at the University of Florida, which uses Fusion F400s, F306s, Lulzbot Taz6, and Ultimaker 3 Extended 3D printers. The printers also offer options for different materials, such as ceramics and metal. The digital squeeze is imported into the 3D model software and printed as an object. This “new artifact” preserves the information that the digital squeeze holds but can be touched, read closely, and consequently bring more unique and authentic experiences to the student and the scholar, beyond what a 2D image or even a digital copy can effect.28
Finally, within this scope, our project has begun utilizing virtual and augmented reality head-mounted displays, such as Meta Quest and Microsoft’s HoloLens headsets. The interface allows the users to browse through 3D databases of artifacts and visualize them within their actual physical space. Such uses of the available technology do not interfere with the integrity of the field of study or undermine scholarly rigor. Au contraire, scholars and students showcase the evolution of the discipline and enhance their research. Standardizing technologies for extended reality, such as openXR and webXR, has enabled immersive visualizations within web applications such as our web 3D viewer. Visualizing a 3D digital squeeze in virtual and augmented reality allows the users to naturally interact with them within their physical space and perform various tasks intuitively.
Digitization, 3D printing, and augmented reality that allow for virtual reconstructions of ancient sites and artifacts do not simply reignite the public’s interest but also allow every new generation to develop personal connections with the artifacts and contribute to their study and understanding, henceforth to their preservation. The DEA project aims to preserve squeezes and other historical artifacts and envisions democratizing their use. We believe that our methodologies, approaches, and worldview will result in the appreciation of world heritage, not as a mandate for the few but as an exigency for everyone. Ultimately, we hope to turn the concept of world heritage into one world heritage and our heritage.
Conclusion
In closing, the overall mission of the DEA is to fulfill the potential to further a diverse audience’s understanding of historically significant inscribed texts and promote new modes of research and teaching that embrace the multidimensionality of ancient artifacts. Such approaches will help to contemporize the discipline, engage a multidisciplinary scholarly audience, and hopefully serve the interests of communities around the world. In The Library at Night, Albert Manguel said:
“We can roam the bloated stacks of the Library of Alexandria, where all imagination and knowledge are assembled; we can recognize in its destruction the warning that all we gather will be lost, but also that much of it can be collected again; we can learn from its splendid ambition that what was one man’s experience can become, through the alchemy of words, the experience of all, and how that experience, distilled once again into words, can serve each singular reader for some secret, singular purpose.” (p. 33)
Creativity filtered through and assisted by technology should have an impact on the historical record. World heritage, both in terms of artifacts and texts, is not only a concern, prerogative, or object of study for epigraphists, archaeologists, historians, humanists, and artists. It should be an integral part of the human experience; it should be re-studied, re-lived, and re-experienced so that it may preserve the past, better the present, and guarantee the future. Digital preservation projects can enrich arts and humanities research and move them beyond the academic sphere in an attempt to: quoting UNESCO, “maintain, increase, and diffuse knowledge by assuring the conservation and protection of the world’s heritage.”29
References
- Abrahamson, D. and Lindgren, R. (2014): “Embodiment and embodied design”, in: Sawyer, ed. 2014, 358-376.
- Alibali, M. W. and Mitchell, J. N. (2009): “Teachers’ gestures as a means of scaffolding students’ understanding: Evidence from an early algebra lesson”, in: Goldman, R., Pea, R., Barron, B., and Derry, S. J. ed.: Video Research in the Learning Sciences,New York, 349–365.
- Amin, M., Barmpoutis, A., Berti, M., Bozia, E., Hensel, J. and Naether, R. (2018): “Depth map of the Rosetta Stone”, Humanities Commons, 1-1 http://dx.doi.org/10.17613/t1e2-0w02 (accessed October 31, 2023).
- Amin, M., Barmpoutis, A., Berti, M., Bozia, E., Hensel, J. and Naether, F. (2023): “The Digital Rosetta Stone Project”, in: Lucarelli, R., Roberson, J. A. and Vinson, S. ed.: Ancient Egypt, New Technology: The Present and Future of Computer Visualization, Virtual Reality and other Digital Humanities, Harvard Egyptological Studies 17, Leiden-Boston, 58-84.
- Antonetti, C., Matijašić, I., De Vido, S. and Mignosa, V. (2017) : “Digital Epigraphy at the Greek Epigraphy Laboratory, Ca’ Foscari University of Venice”, Historika, 7, 491-502.
- Barmpoutis, A., Bozia, E. and Wagman, R. (2010): “A novel framework for 3D reconstruction and analysis of ancient inscriptions”, Journal of Machine Vision and Applications, 2, 6, 989-998.
- Barmpoutis, A. (2013): “Digital Epigraphy Toolbox”, Humanities Commons, 1-11 http://dx.doi.org/10.17613/M64W9R (accessed October 31, 2023).
- Barmpoutis, A. and Bozia, E. (2017): “Augmenting the Workspace of Epigraphists. An interaction design study”, in: Orlandi, S., Santucci, R., Mambrini, F. et Liuzzo, P., ed.: Digital and Traditional Epigraphy in Context: Proceedings of the EAGLE 2016 Conference, Collana Convegni 36, 209-220.
- Benjamin, W. (2010): “The Work of Art in the Age of Mechanical Reproduction”, in: Gale, M., Deeney, J. and Rebellato, D. ed.: Routledge Drama Anthology and Sourcebook, London, 445-468.
- Bozia, E. (2018): “Ektypa and 3D models of ektypa: the reality(ies) of a digital object”, in: Di Giuseppantonio Di Franco, P., Galeazzi, F. and Vassallo, V. ed.: Authenticity and cultural heritage in the age of 3D digital reproductions, Cambridge, 97-110.
- Bozia, E. (2023): “‘Please, touch the exhibits’: 3D archaeology for experiential spatialization”, in: Landeschi, G. and Betts, E. ed.: Capturing the Senses, Digital Methods for Sensory Archaeologies, New York.
- Carver, E. (1989): Cathedral, New York.
- De Jonge, C.C. (2008): Between Grammar and Rhetoric, Leiden-Boston.
- Eisenberg, M. and Pares, N. (2014): “Tangible and full-body interfaces in learning”, in: Sawyer, ed. 2014, 339-357.
- Elia, R.J. (1997): “Looting, collecting, and the destruction of archaeological resources”, Nonrenewable Resources, 6, 85-98.
- Ferrari, G.R.F. (1989): “Plato and Poetry”, in: Kennedy, ed. 1989, 92-148.
- Ford, S. and Minshall, T. (2019): “Where and how 3D printing is used in teaching and education”, Additive Manufacturing, 25, 131-150.
- Gallen, R., Eastop, D., Bozia, E. and Barmpoutis, A. (2015): “Digital imaging: the application of shape-from-shading to lace, seals and metal object”, ICON Journal of Conservation, 38, 1, 41-53.
- Goldin-Meadow, S. (1999): “The role of gesture in communication and thinking”, Trends in cognitive sciences,3, 11, 419-429.
- Goldin-Meadow, S. (2009): “How gesture promotes learning throughout childhood”, Child Development Perspectives, 3, 106-111.
- Halliwell, S. (1989): “Aristotle’s Poetics”, in: Kennedy, ed. 1989, 149-183.
- Kennedy, G., ed. (1989): The Cambridge History of Literary Criticism, Cambridge.
- Levivier, A., Leblanc, E. and Brunet, M. (2016): “E-STAMPAGES : archivage et publication en ligne d’une ectypothèque d’inscriptions grecques”, Les nouvelles de l’archéologie, 145, 24-27 https://journals.openedition.org/nda/3801 (accessed October 31, 2023).
- Lindgren, R. and Johnson-Glenberg, M. (2013): “Emboldened by embodiment: Six precepts for research on embodied learning and mixed reality”, Educational Researcher,42, 8, 445-452.
- Manguel, A. (2009): The Library At Night, Yale-New Haven.
- Meskell, L. (2018): A Future in Ruins: UNESCO, World Heritage, and the Dream of Peace, Oxford.
- Montaner, J.M. (1990): New Museums, London.
- Montusiewicz, J., Barszcz, M. and Korga, S. (2022): “Preparation of 3D Models of Cultural Heritage Objects to Be Recognised by Touch by the Blind—Case Studies”, Applied Sciences, 12, 23, 11910.
- Neely, L. and Langer, M. (2013): “Please feel the museum: The emergence of 3D printing and scanning”, in: Proctor, N. and Cherry, R. ed.: Museums and the Web, Sliver Spring https://mw2013.museumsandtheweb.com/paper/please-feel-the-museum-the-emergence-of-3d-printing-and-scanning/ (accessed October 31, 2023).
- Pearson, H.A. and Dubé, A.K. (2022): “3D printing as an educational technology: theoretical perspectives, learning outcomes, and recommendations for practice”, Educ Inf Technol, 27, 3037-3064.
- Ridgeway, B.S. (1984): Roman Copies of Greek Sculptor, Michigan.
- Rossetti, V., Furfari, F., Leporini, B., Pelagatti, S. and Quartam, A. (2018a): “Enabling Access to Cultural Heritage for the visually impaired: an Interactive 3D model of a Cultural Site”, Procedia Computer Science, 130, 383-391.
- Rossetti, V., Furfari, F., Leporini, B., Pelagatti, S. and Quarta, A. (2018b): “Smart Cultural Site: an Interactive 3d Model Accessible to People with Visual Impairment”, IOP Conf. Ser.: Mater. Sci. Eng., 364, 12-19.
- Sawyer, R., ed. (2014): The Cambridge handbook of the learning sciences, Cambridge.
- Sharpe, S. (1871): The Rosetta Stone, in Hieroglyphics and Greek; with Translations, and an Explanation of the Hieroglyphical Characters; and followed by an Appendix of Kings’ Names, London.
- Swain, S. (1996): Hellenism and Empire, Oxford.
- Themistocleous, K., Agapiou, A. and Hadjimitsis, D. (2016): “Experiencing Cultural Heritage Sites Using 3D Modeling for the Visually Impaired”, in: Ioannides, M., Fink, E., Moropoúlou, A., Hagedorn-Saupe, M., Fresa, A., Liestøl, G., Rajcic, V. and Grussenmeyer, P. ed.: Digital Heritage. Progress in Cultural Heritage: Documentation, Preservation, and Protection. EuroMed 2016, Information Systems and Applications, incl. Internet/Web, and HCI 10059, 171-177.
- Vernant, J.P. and Zeitlin, F. I., ed. (1991): Mortals and Immortals: Collected Essays, Princeton.
- Whitmarsh, T. (2001): Greek Literature and the Roman Empire, Oxford.
Notes
- See Anguissola, Anna “Greek Originals and Roman Copies”. In obo in Classics, https://www.oxfordbibliographies.com/view/document/obo-9780195389661/obo-9780195389661-0213.xml (accessed 19 Jan. 2023). See also Ridgeway (1984).
- For a full discussion of mimēsis in Plato and Aristotle, see Ferrari 1989, 108-141, Halliwell 1989, 151-164, Vernant & Zeitlin 1991, 164-185.
- van Groningen (1965) failed to appreciate the works of the Second Sophistic, and his views found proponents in the work of Bowersock (1969) and Perry (1955). On the other hand, De Jonge (2008), Swain (1996), Whitmarsh (2001), and others reappreciated the creativity in the imitative practices of the period.
- For a detailed discussion of authenticity in digital epigraphy, see Bozia 2018.
- See Meskell (2018) for a thorough discussion on the significance of and perils threatening world heritage as well as the history of organizations working towards its protection. See also Elia 1997.
- https://www.theguardian.com/artanddesign/jonathanjonesblog/2016/apr/06/digital-rembrandt-mock-art-fools (accessed January 30, 2023).
- https://www.businessinsider.com/3d-printed-works-of-art-for-the-blind-2016-1 (accessed January 30, 2023).
- See Rossetti et al. (2018a, b) who detail their use of 3D models to increase the accessibility of architectural details for the visually impaired. See also Montusiewicz et al. 2022, Themistocleous et al. 2016.
- Barmpoutis 2013.
- Grant HD-51214-11 for the Digital Epigraphy Toolbox. Office of Digital Humanities, National Endowment for the Humanities https://securegrants.neh.gov/publicquery/main.aspx?f=1&gn=HD-51214-11 (accessed January 30, 2023).
- Bozia 2018.
- Levivier 2016.
- Antonetti 2017.
- Barmpoutis et al. 2010.
- For the depth map and the normals, see, in this volume, Comte & González Bordas, 19 and 16, n. 25 respectively.
- Barmpoutis & Bozia 2017.
- V
- Our open-source software can also be installed within the web infrastructure of other institutions in order to accommodate local or national mandates related to digital data sovereignty.
- Amin et al. 2023.
- Amin et al. 2018.
- 3D Model of the Rosetta Stone by Jon Beck https://www.myminifactory.com/object/3d-print-rosetta-stone-at-the-british-museum-london-4537 (accessed January 30, 2023).
- 3D Model of the Rosetta Stone by the British Museum https://sketchfab.com/3d-models/the-rosetta-stone-1e03509704a3490e99a173e53b93e282 (accessed January 30, 2023).
- High-contrast readable photograph of the Rosetta Stone. Attributed to the European Space Agency https://upload.wikimedia.org/wikipedia/commons/c/ca/Rosetta_Stone_BW.jpeg (accessed January 30, 2023).
- https://www.britishmuseum.org/collection/image/6109003 (accessed January 30, 2023).
- Amin et al. 2018.
- Sharpe 1871.
- On tactility and re-embodiment both as theories and in archaeological practice, see Bozia 2023.
- See Ford & Minshall (2019) and Pearson & Dubé (2022) who discuss 3D printing as an educational technology. Also, Neely and Langer (2013) present the contributions of 3D printing to the engagement with and embodiment and understanding of the artifact to enhance museum experiences.
- https://whc.unesco.org/en/conventiontext/ (accessed January 30, 2023).